Bioelectricity
Electricity fundamentals for BI360
Electricity is the movement of charged particles. That simple explanation applies to electricity in our daily lives, to currents that flow through wires in the wall, through devices in our hands, as well as cells in our body. The simplest charge carriers are subatomic particles, discovered toward the late 19th century. However, charge can also be carried by atoms, molecules, as well as biologically relevant macromolecules such as proteins and nucleic acids. In fact, animals and even humans can carry a charge in the form of static electricity, which is how we can shock each other, doorknobs, or other metal objects. In this chapter, we will try to understand what charge is, how subatomic particles carry charge and yet create neutrally charged atoms or elements, and finally, how charged particles can be created from neutral atoms or molecules.
Already know all about protons and electrons? Skip to ‘What is Electricity‘ section below!
Electrical charge
What is electrical charge? To answer that, we must travel to the golden age of physics, when the structure of matter was discovered. Physicists in the 19th century thought that matter was comprised of small particles, which couldn’t be divided using the amounts of energy that was available at the time. These particles were said to be indivisible and were named atoms. However, it quickly became apparent that atoms were in fact divisible, if enough energy was somehow applied, and two of the subatomic particles resulting from breaking up an atom carried a force that could either repel or attract. Particles of the same type repelled each other, while particles of two different types attracted each other. The property that these sub-atomic particles carried was named a ‘charge‘, and the two types of charge were named ‘positive‘ and ‘negative. It’s really important to understand that the nouns ‘positive’ and ‘negative’ are only important in that they mean the two kinds of particles have opposing properties, but the ‘positive’ and ‘negative’ doesn’t have an inherent physical implication: they could just as easily have been flipped around, called ‘north’ and ‘south’, or ‘left’ and ‘right’ and so on. The words positive and negative are just convention of usage, and can sometimes be confusing.
Subatomic particles that carried the positive charge were called protons and particles that carried a negative charge were called electrons. These charged particles could be separated separated by supplying enough power, for example, artificially in particle accelerators. Charged particles could were also created in nature: many radioactive materials, like uranium, were known to emit high energy charged particles without human intervention. Ernest Rutherford named the first two types of radioactive emissions as α-rays, which were positively charged, and β-rays, which were negatively charged. How the power in radioactive materials was harnessed by humans is the story of the Manhattan Project, and the subject of a recent movie (Oppenheimer). Scientists also found two other, interesting facts: the first is that the amount of charge on a proton is exactly equal but opposite to the charge on an electron! So when the number of electrons and protons is equal, as in most atoms in nature, these charges cancel out perfectly, resulting in a neutral substance. The other fact, shown in Fig E1, is that the mass of a proton is much, much more than that of an electron.
How were these particles distributed in an atom? The popular model of the atom toward the end of the 19th century, called the plum pudding model, held that held that the two types of charged particles were mixed in equal proportions through the atom, sort of like plums and raisins in a pudding, as shown in Fig E1-A. Ernest Rutherford thought of an interesting experiment to test this idea: he fired high energy α-rays (positively charged) toward a thin film of gold, which is an extremely malleable metal. If the plum pudding model of an atom was correct, then electrons and protons would be mixed uniformly in an atom and the high energy positively charged particles in α-rays would pass right through the film of gold, since individual protons spread through the atom would not be able to repel the high energy α-particles. What did Rutherford actually find?
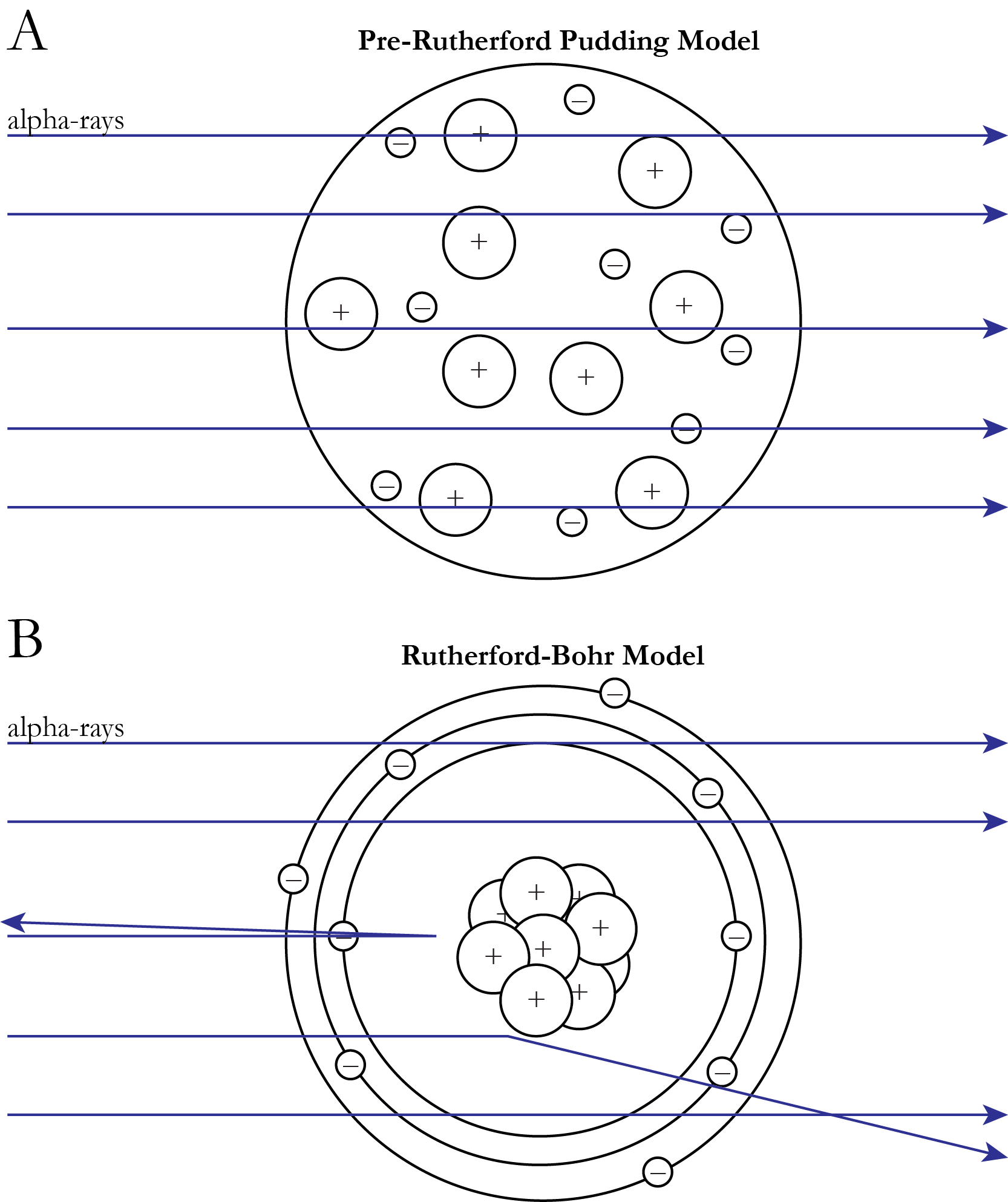
Rutherford found that the vast majority of α particles passed right through the gold film (Fig E2-B), as if most of the atom was empty! Some however were deflected off their path, and even fewer were reflected right back, indicating that somewhere in the atom, there was a concentrated region of positive charges. This result led to the Rutherford-Bohr model of the atom, in which all the positive charges are concentrated in a small volume of space he called the nucleus, while the rest of the atom is empty space (Fig E2-B). Where were the electrons? Orbiting the nucleus, somewhat like planets around the sun: remember, the mass of electrons is negligible compared to that of a proton. This model explained how most α particles passed through the film: an atom was in fact mostly empty space! Subsequent experiments have confirmed that all the protons and neutrons are concentrated in the small volume of the nucleus, while electrons orbit the nucleus at fixed distances, arranged in concentric spheres called ‘orbitals’. Matter in general is neutrally charged, because for each atom, the number of protons is equal to the number of electrons. The simplest element, hydrogen, has one proton in its nucleus, and one orbiting electron. Adding protons and electrons to atoms leads to the formation of new elements[1]; in all elements other than hydrogen, neutrons are also present in nuclei to ensure that the repulsion between protons doesn’t rip the nucleus apart.
So: protons and electrons make up atoms (with neutrons), and in nature, elements have equal numbers of each. How do we go from neutral elements, with equal numbers of electrons and protons, to charged particles?
One way we discussed above was by splitting an atom, which released so much energy that the parts of the atom flew apart, yielding electrons as β-rays, and positively charged α-rays: alpha particles carry two positive charges, and are actually made up of two protons and two neutrons — in essence, they are helium atoms.
We can also create charged particles by removing or adding electrons to atoms, creating ions. Electrons that are furthest away from the nucleus are easiest to strip away from the atom. Metals like sodium and calcium are a good example of such elements, which become positively charged in water, by losing one or more electrons. Remember, to start with, the number of protons is equal to the number of electrons. When an atom loses an electron, it assumes an overall positive charge. Other elements form negatively charged ions. Is the negative charge a consequence of losing protons? Well, protons, since they are located in the center of the atom, are really difficult to remove – it’s only in the immensely energetic nuclear reactions that protons can be removed from the nucleus. So how do we get negatively charge ions in everyday life? Some elements have the ability to accept electrons easily: these are said to be electronegative, and tend to form negatively charged ions in water – for example, chlorine. Keep in mind that losing or gaining an electron makes the ion charged, so removing an additional electron from a positively charge ion, or adding an electron to an already negative ion, is extremely energy intensive. We can also form ions from molecules: some molecules can more easily lose electrons, while others can more easily gain electrons.
Macromolecules like proteins can gain electrons in one part of their structure, and lose electrons in another part, forming negatively or positively charged domains.
So: atoms are made up of positively charged protons, and negatively charged electrons. Protons are all located at the center of the atom, and are thus really difficult to extract from an atom; electrons orbit the nucleus at large distances, and thus are easier to extract from an atom. There are also a third kind of subatomic particle called neutrons, which carry no electrical charge, but lets not talk about them!
Charged particles
In the introduction above, we learned about two kinds of charged particles: sub-atomic particles, and ions. The fundamental charged particles are protons and electrons. We also learned that when in water, some atoms lose electrons to form positively cations, while others accept electrons to form negatively charged particles called anions. Charge is represented by the symbol Q, is measured using the unit Coulomb: one Coulomb (1 C) of charge is created by the loss or gain of 6.25 x 1018 electrons. Or to put it differently, 1 C is equal to 6.25 x 1018 charged particles, whether subatomic or not (Fig E.3).
Some particles can carry more than one unit of charge: alpha particles carry two positive charges. More complex ions can carry not just multiple charges of the same type, but also mixed charges – amino acids are the best example of a molecule that simultaneously carries at least a positive (amino) and a negative (acid) charge. Macromolecules like proteins and nucleic acids carry a lot of charge; overall, nucleic acids, which are huge molecules, carry negative charges, and are responsible for maintaining the charge balance across the cell membrane – we will discuss this in class, when we talk about membrane potential.
What is electricity?
Electricity is the movement of charged particles. Simple, right? Yes, and no!
In the modern world, electricity is part of our lives – electrical currents travel along metal wires, because metals, remember, have the ability to lose electrons easily. These loosely attached electrons whiz around wires, carrying current. That’s why electrical cables are made of metals like copper, aluminum, and silver.
In biology, we deal with reactions involving substances dissolved in water – or as we scientists call it, aqueous solutions. Here, electricity is carried largely by ions. However there are special cases where the charges that carry electricity are subatomic particles: for example, the Electron Transport Chain in mitochondria of all living organisms involves the movement of – you guessed it right – electrons! Similarly the enormous amounts of ATP we use is generated by the movement of protons. Wait – didn’t we say that protons are really hard to extract from a nucleus? How then do we have trillions of them floating around in our bodies?
It turns out that of all the elements that can easily lose electrons – usually metals – there is a special case: hydrogen. Hydrogen is the simplest element with the simplest atom: one proton and one electron (Fig E4). That’s it. So when hydrogen loses its only electron, only a proton remains!
Current, resistance, and voltage
Current
The collective movement of charged particles is called current. By convention, current moves in the direction of net movement of positive particles. In Figure E.5A, the movement of positive charges from left to right causes current to move from left to right. However, the movement of negative charges from left to right in Fig E.5B implies that by convention, current in fact moves from right to left! Current is measured using the unit Ampere: one Ampere (1 A) is the movement of one Coulomb of charge through any location. In household electrical wires, the charge carriers are electrons that separate easily from the outermost orbital of metals like copper. When an electrical appliance is required to do more work – for example, heat up food – more current is required, and more flows through electrical cables.
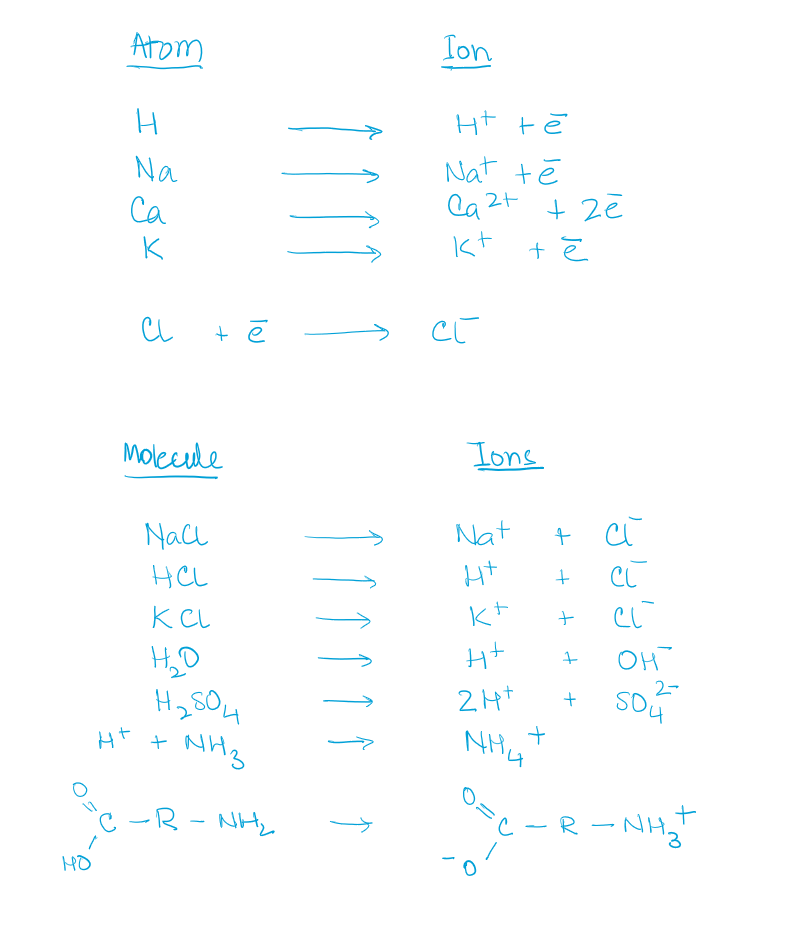
In the biological context, all reactions occur in an aqueous solution – here, the charge carriers are ions that are created when one atom donates electrons to another. Elements like oxygen and chlorine that can easily accept electrons are said to be highly electronegative. Elements like hydrogen, sodium, potassium and calcium, that easily donate electrons, are less electronegative. Dissolving NaCl in water immediately results in the creation of two sets of ions: Na cations, and Cl– anions. These are free to move, for example. to respond to concentrations of positive or negative charges. When these ions move in water, electrical current flows.
Voltage
What makes charges move? Or to put it differently, why does current flow? Voltage, or electrical potential, is the force that causes current to flow and is driven by a difference in charge at one location compared to another location. In biology, voltage is also referred to as the electromotive force (emf). Like gravity causes water to flow from higher to lower levels, voltage causes current to flow from a region of high potential to a region of low potential, and tends to balance out charge differences between the two locations. The SI unit for electrical potential is Volt; one Volt (1 V) is the potential between two places, when one Joule of power is required to move 1 C of charge between the two locations.
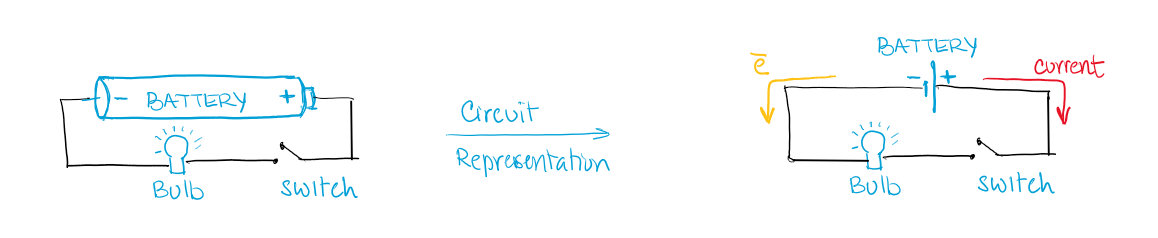
One can see electrical potential most easily in a battery: AA or AAA batteries have a positive end and a negative end, and if you read the description on a battery, you will see that they hold 1.5 V of electrical potential. This potential can be used for work if the two ends are connected. By adding batteries in series, we can create higher potentials (3 V, 4.5 V, 6 V etc.). A simple circuit showing a battery, a light bulb, and a switch are shown in Fig E4. A battery is depicted in FigE4 A, while the typical circuit representation of the battery is shown in FigE4 B. The battery has a positive pole or electrode called the anode, and a negative pole or electrode, called the cathode. By convention, as described above, the direction of current flow is from the positive to the negative pole of the battery (red arrow, Fig E4 B), but in reality, in metal wires, the charge carriers are electrons, which would of course flow in the opposite direction, from the cathode to the anode!
In a fully charged battery, positive charges are accumulated at the anode, and negative charge is accumulated at the cathode. Within the battery, a partition prevents charges from traveling directly to the opposite electrode, which is why we must connect the two electrodes using an external wire. Once we do that, and close the switch, charges will begin to move, causing current to flow. The current flows because the charge is different between the electrodes: this difference is called the electrical potential, or voltage! The bulb will then light up because current is flowing through it. If we keep the circuit running, what do you think will happen to the charge difference between the two electrodes? You can imagine that with every electron that reaches the anode, the two electrodes lose their charge difference. As electrons keep flowing, the battery loses potential, and the ‘voltage’ of the battery declines, until it has no potential left.
In biology, charge carriers are usually ions, created when atoms lose or gain electrons (Fig 3A), or when molecules lose or gain electrons (Fig 3B). Electrical potential is created in biology across semi-permeable membranes, by separating charges on one side of the membrane or another. For example, , in a neuron, there are more positive charges on the outside of the cell membrane compared to the inside. If free to move, positive charges form the outside would enter until the number of charges on either side of the membrane were balanced. In this case, current would flow into the neuron. We will discuss this in Lecture 2, when we talk about membrane potential.
Resistance
Resistance is a measure of the difficulty with which charges can move. When charge move easily, the resistance is said to be low. When it is difficult for charges to move, the resistance is said to be high. A high resistance can occur due to various reasons: the conductive material may be reluctant to free up electrons, the material in which charges must move could be very viscous, or there may be physical barriers to the movement of particles that have very small channels, so that charges can only move through a few at a time rather than in a flood. Resistance is measured with the unit Ohms (Ω). One Ohm (1 Ω) is the resistance between two points where 1 V of potential can move 1 A of current between the two points.
In biology, resistance can change with changes in the size and number of pores in the membrane that allow ions to travel. If the pores are small, ions travel with difficulty, and the resistance will be high. However, if the size of the pore is increased, ions will flow more easily, and the resistance will be lower. Resistance also changes with the number of passages that allow ion movement, and with other factors like the viscosity of the fluid.
Conductance
Conductance is simply the inverse of resistance. Initially, even the units were inverted: the unit of conductance was called the Mho, represented by an upside-down sigma. However, these days, the SI unit of conductance is called the Siemen (g), which in magnitude is still the inverse of 1 Ohm. Conductance can be a more convenient when one is describing the ease of movement of ions, rather than resistance; when we talk about the difficulty of ions moving across membranes, we measure resistance; but when we talk about the ability of ion channels or pores to allow ions through, we often measure their conductance.
Equations Regarding Current
- I = Q / t (where I = current; Q = charge; t = time)
- V = E / Q (where V = voltage; E = energy; Q = charge)
- R = V / I (where R = resistance; V = voltage; I = current)
- Can also be expressed as:
- V = I*R
- I = V / R
- I = V x g (since conductance is the inverse of resistance)
- Can also be expressed as:
- Adding subatomic particles (protons, neutrons or electrons) to atoms requires a tremendous amount of energy, and in nature, only happens in the center of stars via fusion. Every element other than hydrogen formed inside a star; so all the elements heavier than H, that make up a human like you (C, O, P, K, Na etc) were formed at the center of stars, then released into the cosmos when stars exploded. These elements in turn became parts of new stars, and fusion added more particles and created even heavier elements, and so on. ↵
Alpha (α) rays are high energy rays emitted by radioactive particles. α-rays are comprised of alpha (α) particles, each of which carries two positive charges. Based on its mass, it was apparent that each α-particle is equivalent to a Helium (He) nucleus, with two protons and two neutrons.
Beta (β) rays are emitted by radioactive materials. β-rays were found to be made up of high energy electrons; so each β-particle is an electron, moving at a high speed because breakdown of radioactive atoms results in emission of electrons which are accelerated to a high speed..
The model of an atom where all subatomic particles are distributed evenly within the atom, like plums, raisins, and nuts in a pudding. Note that by this time, scientists already knew that atoms were made up of subatomic particles, named neutrons, protons and electrons.
Malleability is the property of a metal that allows it to be beaten (hammered) into extremely thin sheets. Gold can be made into sheets that are only a couple of atoms thick! These sheets are so thin, that some are used as decoration in very expensive sweets in South Asia, especially during weddings and festivals.
More electronegative elements have atoms that easily attract or accept electrons. Oxygen is an important biologically relevant electronegative element; in fact, oxygen is the most electronegative biologically relevant element.