47 Memory Systems
Think back to your favorite birthday party. Which of your friends were there? What did you do, where did you go, and did you have cake? Did you get gifts? The ability to perform this task depends on our ability to create and recall memories. According to our current best understanding of the neuroscience of learning, the underlying biology of a memory mainly consists of subtle changes among synapses distributed across several brain areas. Our ability to learn new facts, recount the events of last week, or to perform new motor skills is the result of learning-induced neural plasticity. In our discussion of learning and memory, we will consider different aspects of learning and memory, starting from the behavioral level down to the molecular changes responsible for memory formation.
Types of Memory
First, it is important to distinguish between different types of memories.
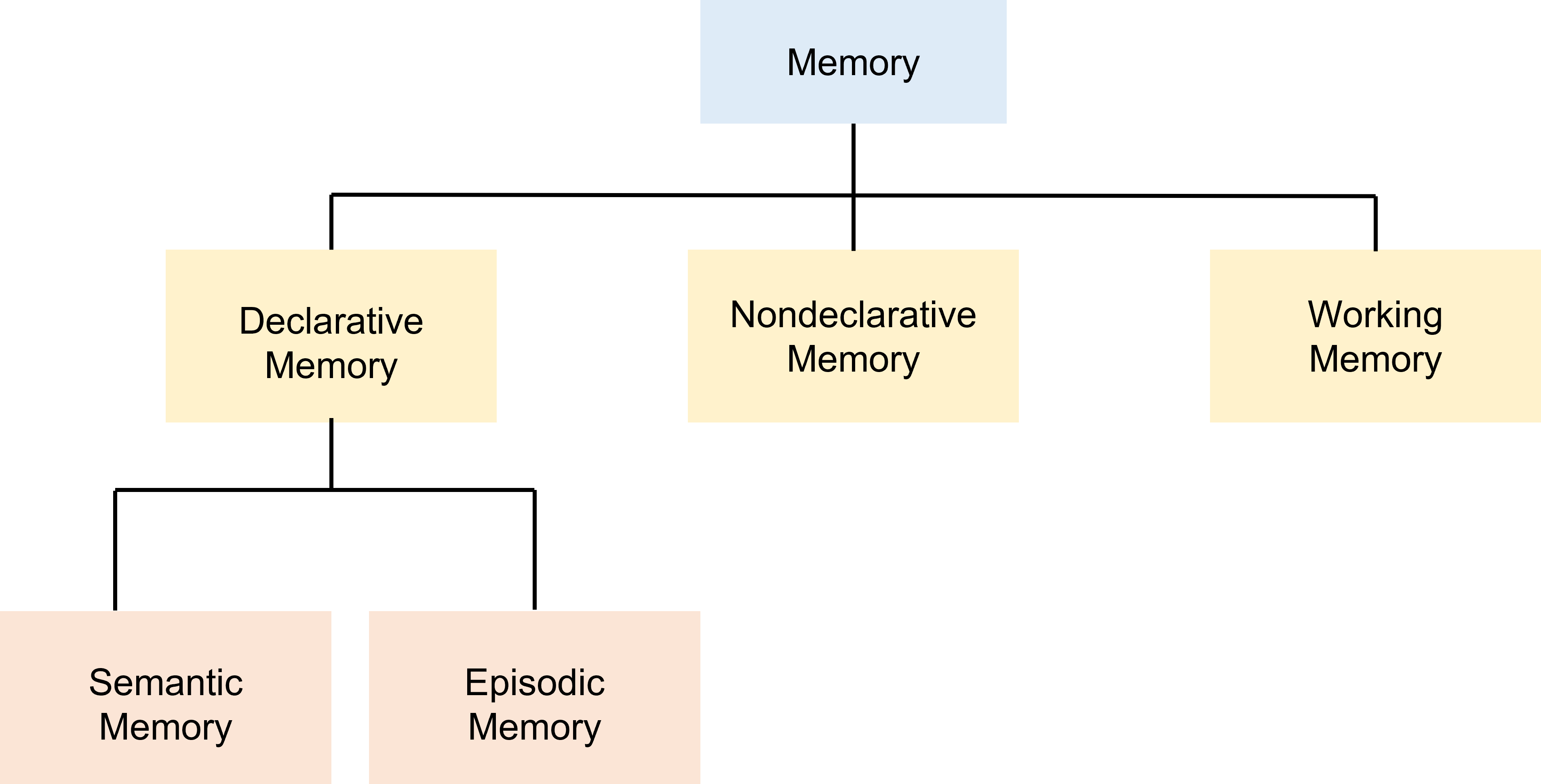
Declarative Memory
Declarative memories, also called explicit memories, are the pieces of information that can be consciously declared or stated explicitly. Declarative memories are thought of as a “knowing what”. Declarative memories can be further subdivided into semantic memory and episodic memory. Semantic memories are pieces of factual information. Some examples include: 1. “Jupiter is the largest planet of our solar system.” 2. “Rosalind Franklin discovered the doublehelix structure of a DNA molecule.” 3. “The actor Keanu Reeves played the protagonist of the movie The Matrix.”
An episodic memory, sometimes also called an autobiographical memory, is the recollection of a discrete moment in a person’s life. It can be thought of as “mental-time travel”—what was it like when. The following memories are examples of episodic memories: “When I got home, I put my wallet and phone on the table.”, “I ordered pizza last night.”, “In 2019, I went to see my favorite musicians perform live.”.
Declarative memories can either be a short-term memory or a long-term memory, which are distinct from each other. The process of memory consolidation takes facts and events and converts them from short-term memory into a long-term memory, which is supported by structural and functional change within the neurons that hold the long-term memory.
Nondeclarative Memory
Nondeclarative memory encompasses all other types of memory outside of semantic and episodic memory including procedural memories, skeletal muscle memory, and emotional response memory.
Procedural memories, (or implicit memories) are unconscious memories, and can’t be explicitly stated. These can be thought of as “knowing how”. Some examples of procedural memories include, for example, performance of a series of motor actions without conscious thought such as an experienced musician playing a simple scale (sometimes commonly called “muscle memory”, even though the muscles do not store any actual memory!), or a priming effect (such as when a person sees pictures of bananas, they are more likely to answer the fill-in-the-blank prompt “b _ _ _ _ _” with “banana”, whereas other people might guess “bubble” or “badger”.
There are two different types of procedural learning: non-associative learning and associative learning.
Non-Associative Learning
Non-associative learning involves a change in behavioral response over time in response to a single type of stimulus and includes habituation and sensitization. In habituation, responses decrease after repeated presentation of a stimulus as the individual learns that the stimulus lacks meaning. For example, if someone poked you in the back, you will initially jump in surprise. However, if someone repeatedly pokes you in the back over and over again, your response will decrease and you realize that the stimulus is meaningless.
In sensitization, responses increase following presentation of a strong stimulus. For example, a sound of a door opening in your house typically will not cause a strong response. However, if you are home alone and hear a loud bang followed by a door opening, you will likely be on high alert.
Associative Learning
Associative memories are the types of information that we learn through traditional Pavlovian conditioning. For example, recall the classic Nobel prize-winning experiment in physiology conducted by Ivan Pavlov in the late 1800s. Normally, the presentation of dog food, an unconditioned stimulus (US), causes a dog to salivate, a naturally occurring behavior, called the unconditioned response (UR). Dogs are not particularly interested in the sound of a bell: this neutral stimulus will produce a minor response, such as a head turn and attentional shift towards the origin of the sound, but not much more than that. However, when this stimulus is repeatedly paired with the presentation of food, dogs quickly learn to associate that the bell signals food. After multiple pairings, upon hearing the bell, a conditioned stimulus (CS), the dogs begin to salivate, a conditioned response (CR), independent of any food being presented.
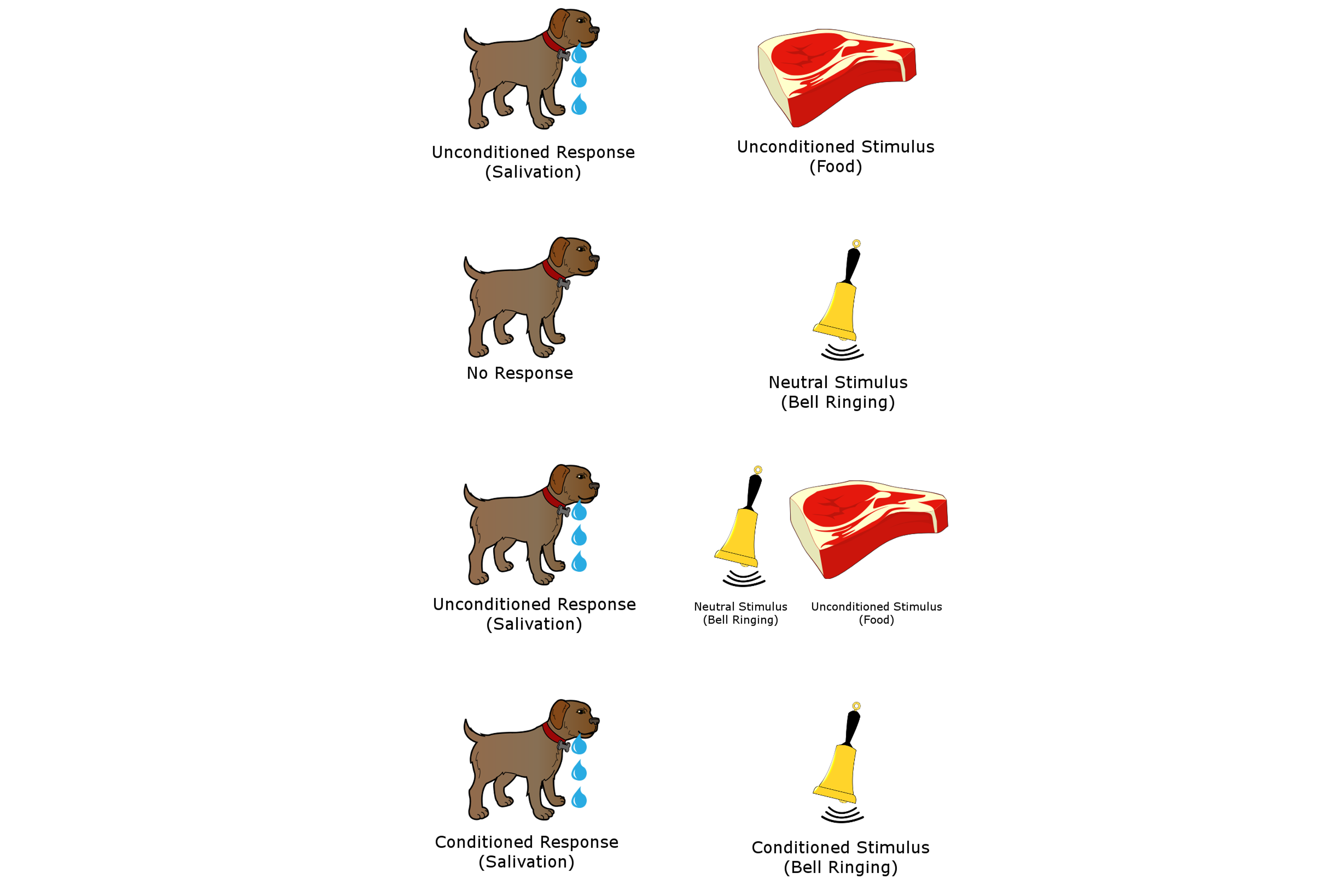
Associative learning can also be measured with instrumental conditioning where a rodent, for example, learns to associate a response with a meaningful stimulus. For example, a rodent will learn to press a lever for access to food.
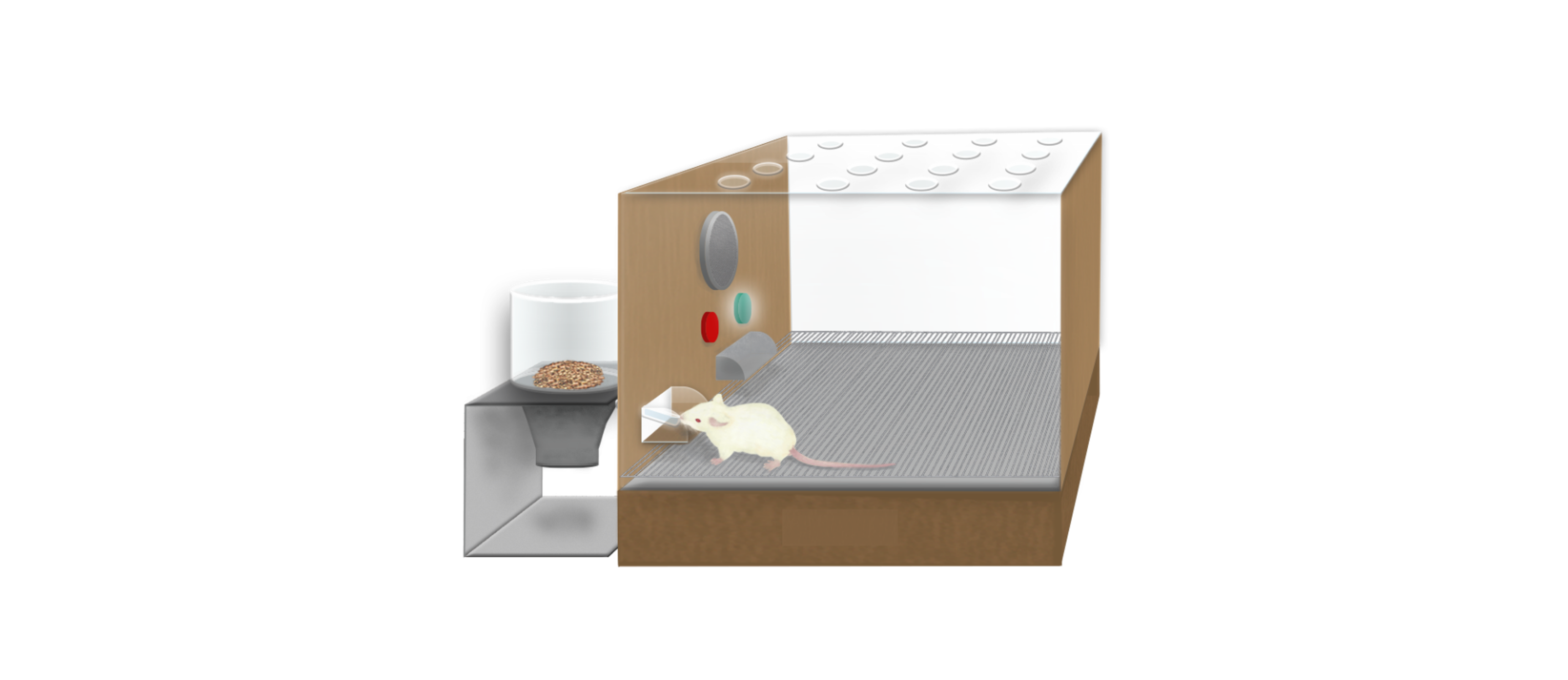
Working Memory
Separate from declarative or procedural memories, a different form of memory called working memory involves processes of storing information temporarily while simultaneously manipulating those pieces of information. This type of memory can be thought of as a “short-term memory on overdrive.” For example, a test of working memory is the digit span test, where a person is given a series of numbers to remember, then they are asked to repeat the numbers in reverse order. After successfully completing this task, a different series of numbers, this time one digit longer, is presented to the patient until they first start making errors in recall. A related task is called the Corsi block tapping test, where an experimenter sets up several blocks on a table. The experimenter then taps a series of blocks in a specific order, then the subject is asked to tap on the blocks in reverse order. As with the digit-span test, the experimenter then makes the series of blocks longer until they make mistakes in the tapping.
Brain Structures in Memory
Declarative Memory—Cortex
In the 1950’s, the scientist Karl Lashley was interested in finding a location in the brain where memories were stored. His experiments searched for the location within the cortex of what he called an “engram” or memory trace. He trained rats to run in a complex maze to reach a food reward, which took the animals time to learn. After the animals had learned how to successfully run the maze, Lashley would lesion different areas of the rat cortex and then test the rats in the maze again. He found that regardless of the location of the lesion, which was done at different areas of the cortex for each rat, that cortical lesions resulted in decreased ability to remember the location of the food reward and run the maze. Instead of finding a specific location where the memories were being stored, Lashley discovered that the size of the lesion corresponded to the amount of memory deficit in the animal, but that the location of the lesion did not have an effect, demonstrating that the engram was widely spread across the cortex.
Memory Consolidation and Spatial Memory—Medial Temporal Lobes, Including the Hippocampus
The main structures of the medial temporal lobes include the hippocampus, the entorhinal cortex, the perirhinal cortex, and parahippocampal cortex. Together, these structures are important in both consolidation and storage of declarative memories.
The hippocampus, meaning “seahorse” in Greek, was named based on its morphology and resemblance to a seahorse. The hippocampus is located along the ventral and medial surface of the brain. The hippocampus is one of the critical structures of the limbic system, a series of subcortical brain structures that are involved in several different complex behaviors, such as emotions and memory. The limbic system is an evolutionarily ancient brain network.
The hippocampus is involved in spatial memories, memories involved in navigation of our surroundings, and the creation of a mental map of our world. Spatial memories are developed when we enter a new building for the first time and we search for a new classroom. We also use our spatial memory whenever we are walking around campus, making our way from one building to another, thinking about the streets you’d need to cross or the buildings you can cut through. While the volume of the hippocampus is not a reliable indicator of the strength of a healthy person’s spatial memory, injury to the hippocampus causes deficits in spatial memory.
Patient HM
One of the most influential case studies in the neuroscience of memory is the story of Patient HM. Since his death in 2008, we now know that Patient HM’s name was Henry Molaison. HM was born in 1926 in a small Connecticut town. In his childhood, HM began having severe seizures, possibly the result of a head injury. In his teenage years, he started having tonic-clonic seizures, the most severe form of seizures that produces a loss of consciousness and convulsions (extreme muscle contraction or extension). In his early adulthood, he was having a tonic-clonic seizure monthly and several minor seizures daily, preventing him from working a normal job or living a normal life, despite taking a cocktail of anti-epileptic medications.
Neurosurgeon William Scoville proposed a “frankly experimental operation” to treat HM. It was known that most epilepsy originates in patches of neurons of the medial temporal lobe, and HM’s epilepsy was typical in this respect. Scoville suggested to surgically resect, or remove, the medial temporal lobe. In 1953, Scoville removed about 8 cm of the medial temporal lobe bilaterally, including part of the amygdala, and notably the hippocampus, the seahorse-shaped structure of the brain. The surgery succeeded at its primary goal: HM’s seizures were less frequent and less severe. However, HM was left with a highly unusual and life-altering side effect: He was unable to create new declarative memories, a memory deficit called anterograde amnesia. For example, he could not remember what he had eaten for lunch just minutes after finishing the last bite. Despite being an avid fan of watching the news, HM couldn’t remember the names or the faces of different celebrities or public figures. It was as if he was permanently living in the present. (In contrast, retrograde amnesia affects the ability to successfully retrieve memory from one’s past.)
However, despite his pervasive memory deficits, HM did not display any deficits in intelligence. His language and speech were unaffected, and word recall was excellent, as he loved completing crossword puzzles and often did so successfully late in life, with only the occasional spelling errors. He could learn to acquire new skills, such as keeping a pen still on a moving circular platform, or a tapping task (these skills are a different form of memory called procedural memory). He was also capable of recalling things from his early childhood, such as geography facts he had learned in elementary school.
The fact that HM’s medial temporal lobe surgery disrupted some types of memories (e.g., memory for facts) while others were still intact (e.g., motor skills) inspired neuropsychiatrists to try to define the different forms of memory. Much of the research was led by Dr. Brenda Milner, who carried out several behavioral tests on HM to figure out what types of memories are dependent on the intact medial temporal lobe and which ones can function without the medial temporal lobe.
Several tests concluded that HM had lost his ability to create new semantic memories. In one such study, HM was asked to determine if a word was made up or real. He was shown words with very old origins, such as “shepherd” or “butcher.” On these words, he performed as well as the control group. When he was shown words that are made up, such as “phlage” or “thweise”, he likewise performed as well as the controls. However, when shown words that were added into the dictionary after his 1953-surgery, such as “granola” or “jacuzzi,” he scored about 50% correct—consistent with guessing at random, as if he never acquired the knowledge that these words have a meaning.
HM was also unable to create autobiographical memories. When asked to recall one of his birthday celebrations as an adult, he wouldn’t be able to give any significant details about the event. Instead, his answers were often vague and generic. One interesting observation was that HM’s memory about details from his childhood were still intact. The inability to recall memories from the past, in this case, from before HM´s surgery, is called retrograde amnesia. Patient HM’s retrograde amnesia was temporally graded, meaning that the farther back you examine, the more complete his memories were. Many of his memories for the two years before his surgery were completely lost, but memories from his youth and teenage years were intact as much as healthy individuals (there is contention about this observation, because HM was taking several anti-epileptic drugs, which may have impacted memory formation.)
From this observation, memory researchers concluded that the medial temporal lobe functions as short-term storage site for memories, but after some years, those memories get relocated to other brain areas outside of the medial temporal lobe. Currently, the scientific evidence suggests that memories are distributed across several networks of cortical and subcortical brain areas.
While HM lost the ability to create new declarative memories, he was still able to maintain a different class of memories, called procedural memories (or implicit memories). The original test of procedural memory conducted by Dr. Brenda Milner was called the mirror tracing task. In this test, the patient is told to draw a third star in between the two stars as quickly as possible without making any mistakes. The challenge is that the tracing is to be done while watching their hand and the star in their reflection in a mirror. Because of these unusual circumstances, completing this task is difficult. But over multiple days of practice, people become better at this mirror tracing task, completing it faster with fewer errors. Improvement on this task indicates that a person is learning or gaining some memory about how to better perform the task. After practicing this mirror tracing task, HM was able to finish drawing the star about ten times faster than when he first began. He improved his performance within each day’s worth of training, and he also improved day-to-day. There is evidence that he maintained these skills up to one year later, despite not having regular training on this task.
Surprisingly, each day Milner examined HM, she would need to reintroduce herself since he forgot who she was. She also had to re-explain what HM was supposed to do in the mirror tracing task. Hence, while HM was unable to form declarative memory about the experiment or the people involved, learning of the procedural memories and motor actions involved in this task remained intact.
Based on the deficits seen in Patient HM and other experimental manipulations of the hippocampus, we conclude that the hippocampus is strongly implicated in the process of declarative memories and spatial navigation. Since some of HM’s memory functions were still intact, such as procedural memories and working memory, it is believed that these functions are independent of hippocampus function.
Tests of Hippocampal Function in Rodents
To test spatial memory behaviorally in nonhuman animals, one test that is regularly used in rodents is called the Morris water maze. In this test, a shallow pool is filled with an opaque liquid, making it difficult to see through. Hidden somewhere in this pool is a clear plexiglass platform, and surrounding the pool are different environmental cues that can be seen from the surface of the water, such as different shapes or colors. The water is deep enough that when a rodent is put into the Morris water maze, they have to swim to stay afloat. The rodents swim around aimlessly until they find the platform, the time it takes for this to happen is recorded, and the trial ends. Over time, the animals learn that the platform is located near certain navigational cues, and on future trials, the animals spend more time near those cues, and the latency to find the platform decreases. When the hippocampus is surgically removed from rodents or inactivated, they perform poorly in the Morris water maze.
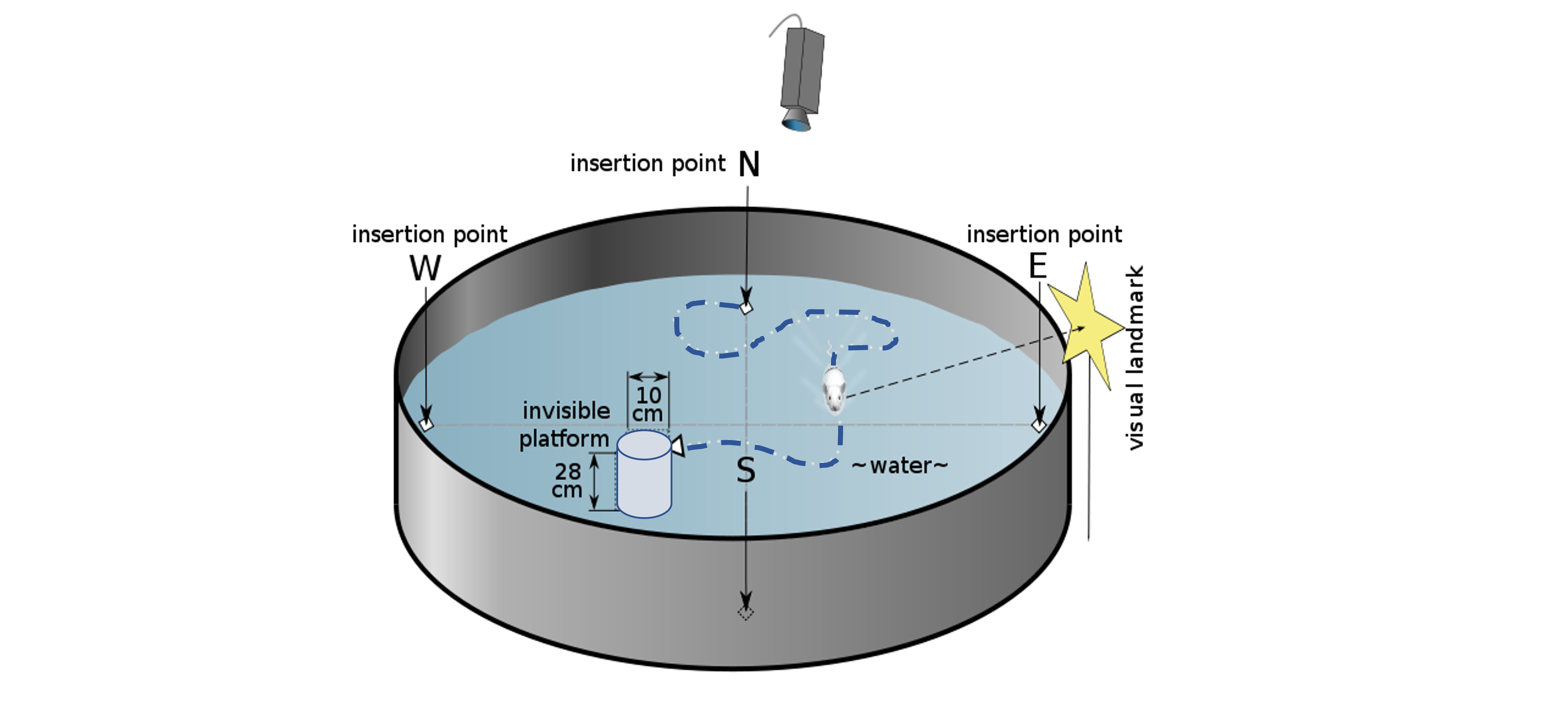
Another non-human behavioral test used to assess the capacity for learning navigational cues is the radial arm maze. In this test, a rodent is placed on a circular platform. Extending from this platform are eight or more “arms”, at the end of each is a small dish. In one of the dishes is a morsel of food (“rewarded arm”), while the other dishes contain nothing (“non-rewarded arms”). The maze is designed so that the food cannot be seen from the end of each arm, so the animal must return to the starting platform before exploring another arm. The number of entries into a non-rewarded arms is counted as an error. Over time, the animals make fewer errors as they learn which arm is rewarded and which ones are not. Alzheimer’s disease model organisms perform poorly on this task.
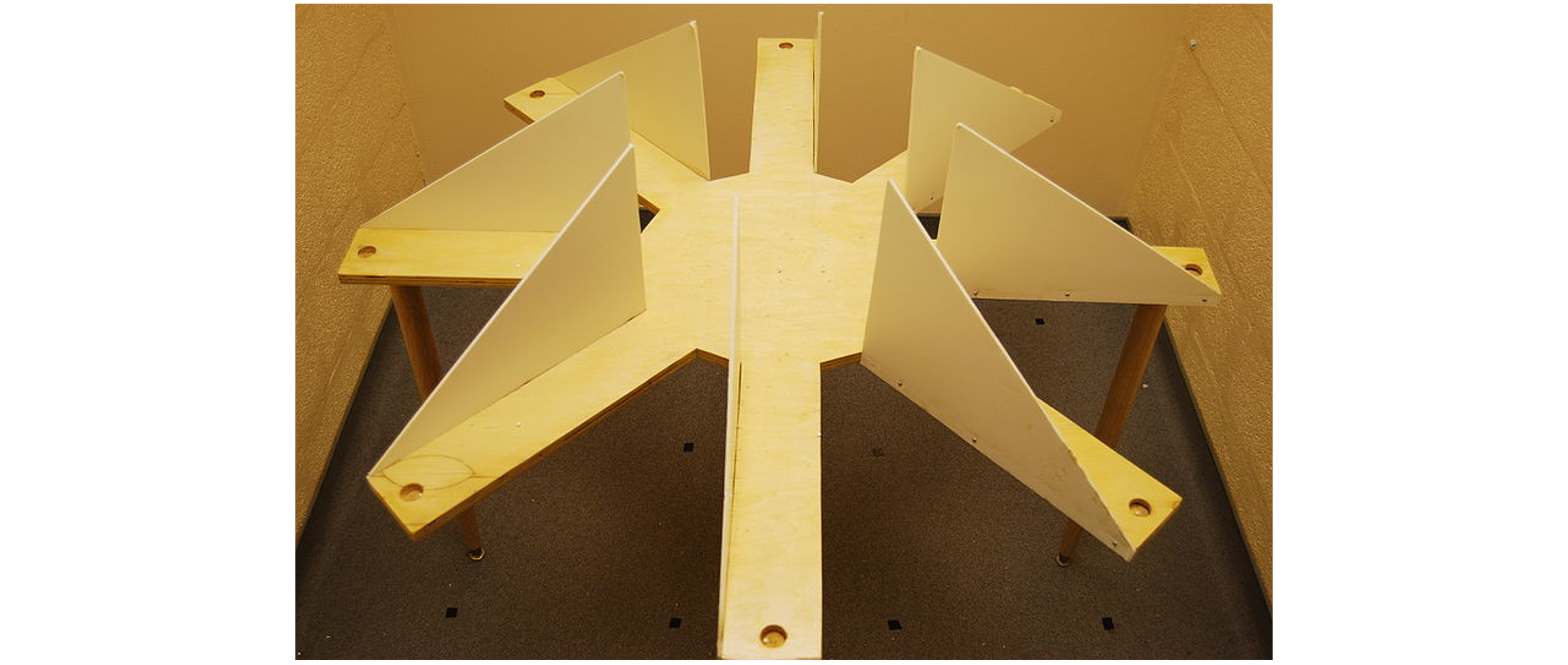
Special Populations of Neurons
An individual memory is likely distributed widely across several different parts of the brain. However, there are a few special populations of neurons mainly in the medial temporal lobe that contribute to highly specific types of memories.
Place Cells
Place cells are a special population of pyramidal cells of the hippocampus. These neurons increase their firing activity when the animal is in a particular location in an environment, indicating that they contribute strongly to location and navigational memory. There is no apparent topographical arrangement of these place cells, meaning that adjacent areas of an environment do not necessarily activate adjacent hippocampal place cells. The place cells, when firing at the right times, help the animal create a spatial map of their surroundings.
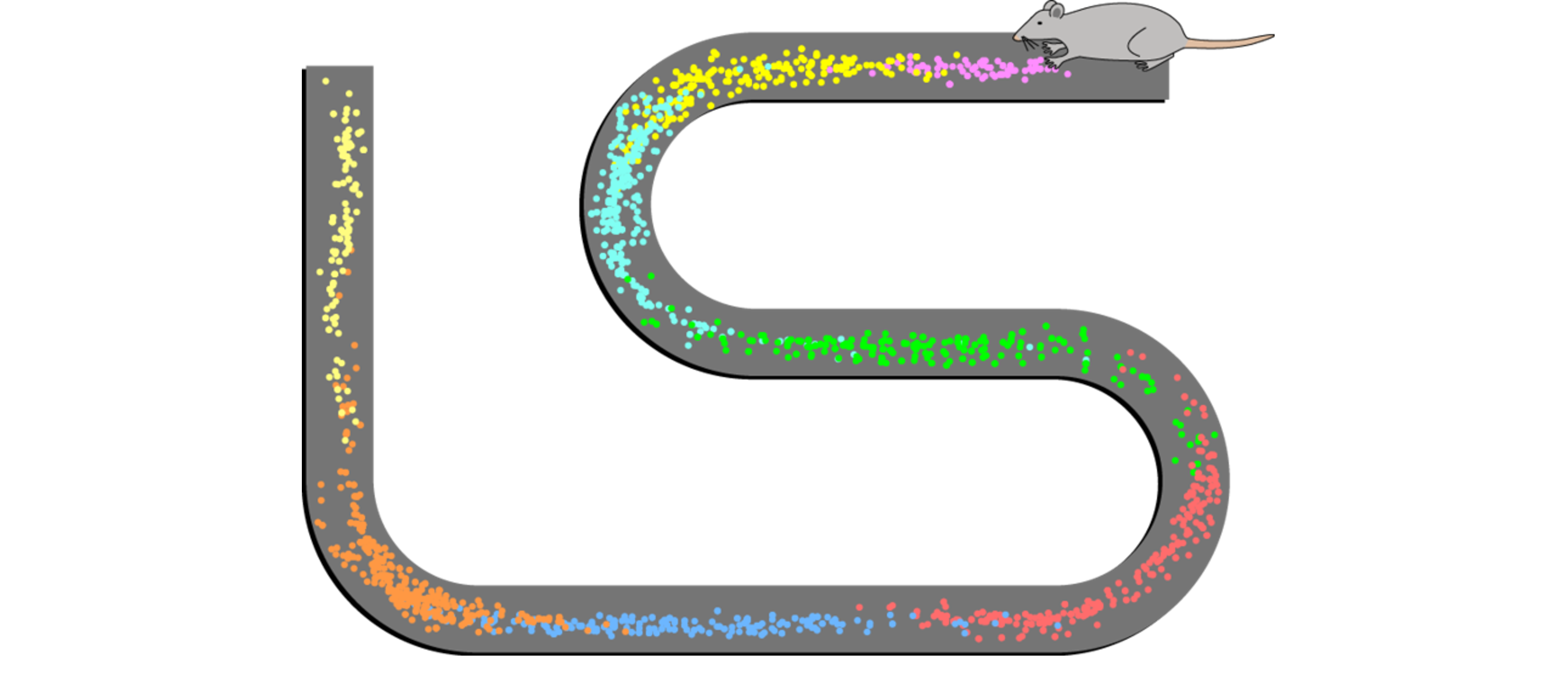
Grid cells
Grid cells are located in the entorhinal cortex, the main input structure to the hippocampus. Closely related to the place cells described above, grid cells increase their firing properties periodically when an animal is at an intersection of a “grid” in a wide-open, previously-explored environment. The grid itself is roughly hexagonal, and spans the whole environment an animal is in. The overlap of multiple grids gives the animal an idea of the surroundings. The scientific description of grid cells earned three scientists, Edvard Moser, May-Britt Moser, and John O’Keefe, a Nobel Prize in Physiology or Medicine in 2014.
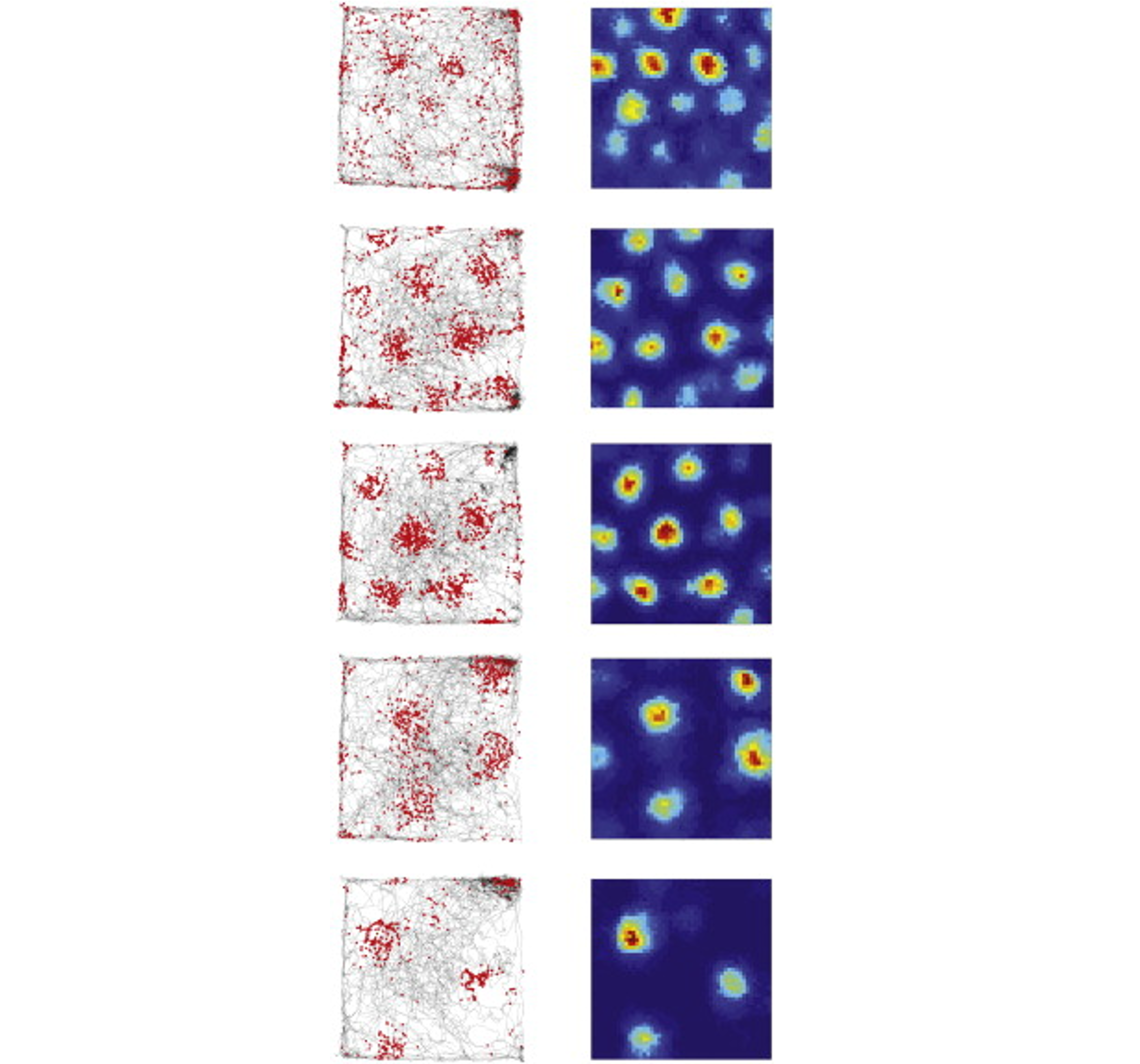
Concept cells
Concept cells are a series of cortical neurons in the temporal lobe that increase their firing exclusively in response to highly-specific stimuli, such as the idea of Jennifer Aniston, Halle Berry, or the Tower of Pisa. These concept cells respond to much more than just pictures: for example, a Luke Skywalker neuron that responded to a picture of young Mark Hamill (the actor who played Luke Skywalker) will also respond to text that reads “LUKE SKYWALKER” and the sound of a person saying “Luke Skywalker”. Although this particular neuron probably won’t fire in response to pictures of athlete Manu Ginobili or actress Marilyn Monroe, the neuron might fire in response to pictures of Yoda or Darth Vader, indicating that the neuron may encode an even broader concept, such as “Star Wars characters” or “Jedi”, or is a part of a network that encodes concepts related to the Star Wars franchise.
Working Memory—Prefrontal Cortex
As part of the frontal lobe, the prefrontal cortex is involved in higher order decision making and personality. In the context of memory, neural circuits in the prefrontal cortex are important for short-term and working memory.
A delayed-response task relies on prefrontal cortex activity. In this task, a monkey is shown a cue of food placed into a randomly selected compartment outside their cage. A screen is then lowered and the compartments are covered for a standard period of time. The screen is then raised again and the monkey is tasked with correctly uncovering the compartment that contains the food. Animals with a lesion to the prefrontal cortex perform worse than animals with an intact prefrontal cortex in this task.
Patients with injuries to their prefrontal cortex after stroke, tumors or aneurysm, performed worse on a variety of working memory tasks such as the digit span test. Additionally, people with frontotemporal dementia, a neurodegenerative disorder characterized by a degradation of the frontal lobe, often have difficulty with working memory. The prefrontal cortex also has strong projections with the hippocampus, and these circuits are likely also involved in the formation of hippocampal-dependent memories.
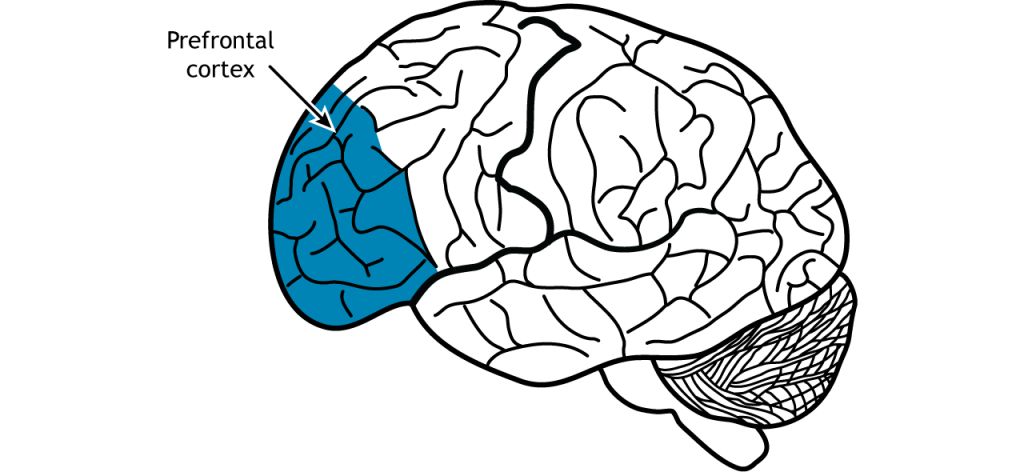
Habit or Procedural Memory—Striatum
The striatum, made up of the caudate nucleus and putamen, is important for habit or procedural memory and reward in learning. A T-maze is used to test procedural learning. In this task, a rodent is placed at the area of the maze that corresponds to the bottom of the T. The animal is trained to turn either left (with a high frequency tone) or right (with a low frequency tone) once they reach the cross of the T. If the animal completes the task successfully, they are rewarded with a treat. The ability of the animal to learn this task is dependent on activity within the striatum. Animals that have striatal lesions have impaired performance on the T-maze, but not on tasks that rely on other types of memory.
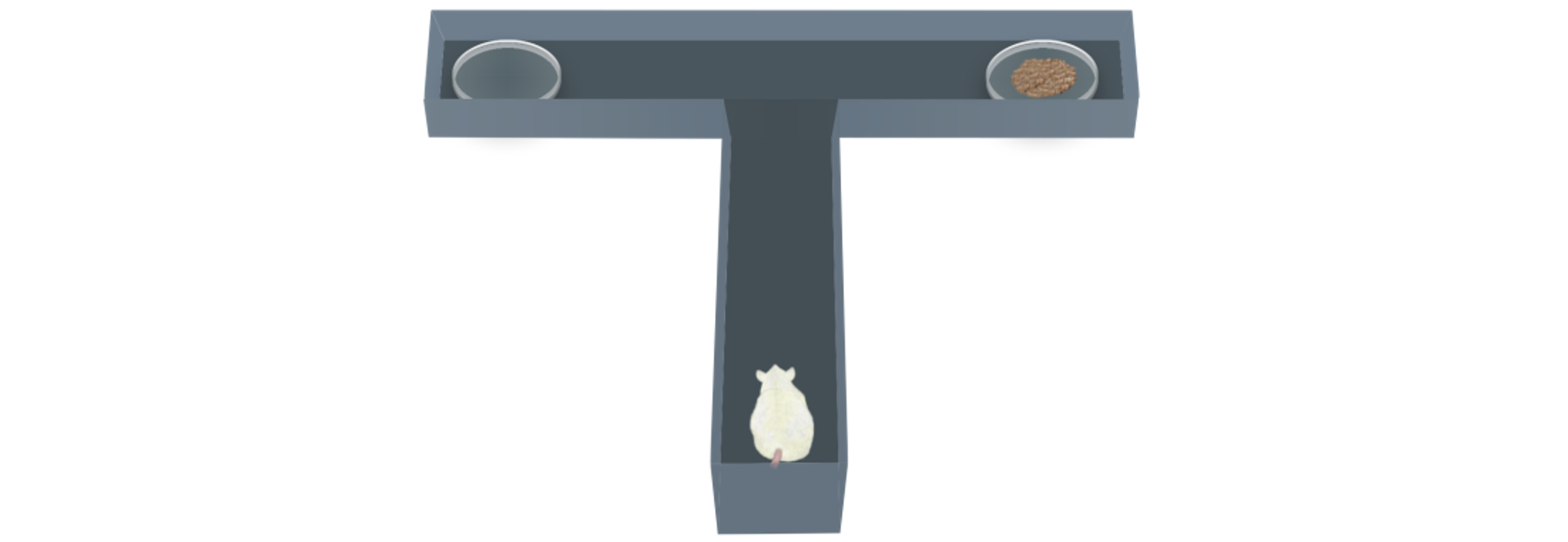
Cellular Memory
In the late 1800s, around the time when Golgi and Ramon y Cajal were engaged in intense debate about the organization of the nervous system, many neuroscientists came to a strange observation: the weight of the brain increases dramatically over the first 10 years of life, but not much more after that. Even though we learn lots of new facts and make lots of new memories in adulthood, the brain itself doesn’t grow in size. So how is it possible to store new knowledge if the brain is not making many new neurons? Most likely, new pieces of information are held in the connections between cells, not just in the cells themselves. If our estimate of 150 trillion synapses per adult brain is correct, then it is possible that we could store all the knowledge and memories that we collect over our lifetime through some combination of activity across certain connections.
The activity at the cellular level is believed to take place on at least three different levels enabling us to build, store, and retrieve memories.
1. Memory Encoding
Encoding refers to the ability for brain circuits to store some piece of information. In real life, you are presented with countless stimuli simultaneously. Imagine walking down a busy street, and think of the number of different sights, smells, and tactile stimuli you experience. Storing memories is an energetically costly process, and we are limited in the fact that all of our sensory inputs cannot possibly get encoded. Instead, evolution has preferred to encode stimuli that are the most salient pieces of information, such as perceptual cues associated with predators. Alternatively, information that we pay strong attention to can get encoded more strongly, like when we repeat a phone number to ourselves until we have a chance to write it down. It is also easier to encode novel information that “builds” on previous bits of knowledge, or information that is closely related to other well-established information, which is why analogies are such an effective way to learn new facts.
2. Memory Consolidation
The process that enables memory storing is called consolidation, which makes the memory more permanent. In 1949, a student of Karl Lashley, the neuropsychologist Donald O. Hebb, offered an explanation for how changes in synapses could possibly lead to a phenomenon as complex as learning. His theory, published in his text The Organization of Behavior, can be summed up in the phrase: “Cells that fire together, wire together.” In Hebb’s framework, repeated activity at a synapse within a circuit of neurons acts as a reinforcer signal that strengthens this synapse for future communication, making the next incoming signal more robust. Hebb also implies the inverse is true: when cells do not fire together, they weaken their connection. Through fine tuning of synaptic connections, some strengthening and others weakening, a lifetime of memories can be stored across a wide distribution of neurons. After a memory has been created, the specific circuit of neurons that represent that piece of information is called a memory trace or an engram. A cellular process called reverberation is thought to be the mechanism that allows for consolidation.
Reverberation is the process by which networks of neurons fire repeatedly. Each time that circuit is activated, the strength of the network is increased, meaning that it becomes easier for that circuit to be activated in the future. Throughout the process of consolidation, memory traces are thought to become more represented in the neocortex and less in the subcortical structures like the hippocampus or amygdala.
Recall Patient HM’s temporally graded retrograde amnesia, where he had lost declarative memories in the two years leading up to this surgery, and yet his memories from the long past were maintained. This finding suggests that some aspects of declarative memory consolidation depends at least partially on medial temporal lobe and hippocampus for a period of time, maybe up to two years, before those memories get stored in the cortex more permanently. However, we cannot conclude how long consolidation takes in healthy humans based on findings from HM, who had pervasive and frequent seizures, which might have negatively impacted memory consolidation before the surgery.
Consolidation seems to occur predominantly during sleep. Specifically, declarative memory is enhanced during non-REM sleep, while procedural memory is enhanced during REM sleep. Studies investigating sleep consolidation are often done by letting participants learn or perform behavioral tasks, after which they are deprived of a specific phase of sleep. To wake participants at the right moment, researchers commonly use EEG signatures, which are unique for different phases of sleep: sleep deprivation early in the night denies non-REM slow wave sleep, while late-sleep deprivation decreases time spent in REM sleep. Some speculate that the hallucinations we sometimes experience during dreaming are a consequence of consolidation processes, but it is inconclusive as to what role dreaming plays in memory formation.
3. Memory Retrieval
Finally, for the stored memories to be recalled, a cellular process called retrieval happens which brings back the specific engram. Retrieval happens for both declarative and procedural memories. The writing of memories can be differentiated from the retrieval of memories using specific priming-related behavioral tests. One example is a vocabulary recall test. Consider if you were given a list of 50 words to memorize, words that belong to a handful of different conceptual categories (such as cinnamon, pepper, or curry, which fall under the category “food flavors”). When asked to write down as many words as possible from the list using your memory, a test called free-recall, you may be able to successfully remember about a third of them. However, if you were prompted with the category titles, a related test called cued-recall, you would perform much better at retrieving those memories, possibly recalling up to 75% of the words. The fact that cued-recall scores are often higher than free-recall scores indicate that there is a distinction between the encoding / consolidation of memories and the retrieval of memories.
Retrieval is not a passive function. When an engram is retrieved, it is reconsolidated, which is an act similar to replaying the activity of the circuit. During this reconsolidation, it is possible that some aspects of the memory are emphasized, while others are lost. This is likely the main reason why we experience false memories, memories that are not true to reality—one reason why eyewitness testimonies are notoriously unreliable. We may imagine our good memories as better than they actually were, while simultaneously dampening the negative aspects of those memories. A dysregulation of this reconsolidation process could lead to the symptoms seen in post-traumatic stress disorder, where the negative emotional components of a particular memory are exaggerated rather than being blunted.
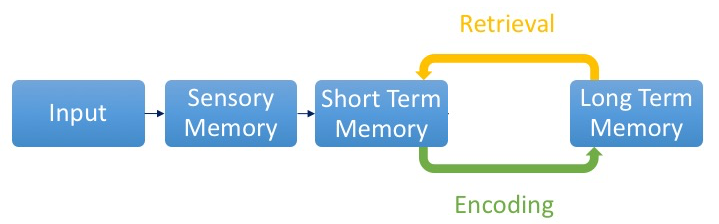
Key Takeaways
- Memory can be divided into declarative memory, nondeclarative memory, and working memory. Declarative memory can be further subdivided into semantic memory and episodic memory.
- The cortex, hippocampus, striatum, and prefrontal cortex are all structures with specific roles in memory.
Attributions
This chapter is adapted from “Memory Systems” in Introduction to Neuroscience by Valerie Hedges which is licensed under a Creative Commons Attribution-NonCommercial-ShareAlike 4.0 International License.
Media Attributions
- Types of memory © Valerie Hedges is licensed under a CC BY-NC-SA (Attribution NonCommercial ShareAlike) license.
- classical conditioning © Salehi.s adapted by Valerie Hedges is licensed under a CC BY-SA (Attribution ShareAlike) license.
- instrumental conditioning © DataBase Center for Life Science (DBCLS) adapted by Valerie Hedges is licensed under a CC BY (Attribution) license.
- Morris water maze © Samueljohn.de adapted by Valerie Hedges is licensed under a CC BY-SA (Attribution ShareAlike) license.
- radial arm maze © Mcole13 adapted by Valerie Hedges is licensed under a CC BY-SA (Attribution ShareAlike) license.
- Place cells © Stuartlayton adapted by Valerie Hedges is licensed under a CC BY-SA (Attribution ShareAlike) license.
- Grid cells © Lisa M. Giocomo, May-Britt Moser, Edvard I. Moser, adapted by Valerie Hedges is licensed under a CC BY-SA (Attribution ShareAlike) license.
- PrefrontalCortex-1024×472-1 © Casey Henley adapted by Valerie Hedges is licensed under a CC BY-NC-SA (Attribution NonCommercial ShareAlike) license.
- T maze © ataBase Center for Life Science (DBCLS) adapted by Valerie Hedges is licensed under a CC BY (Attribution) license.
- Encoding_and_retrieval_image © Mcmactavish adapted by Valerie Hedges is licensed under a CC BY-SA (Attribution ShareAlike) license.
memory that involves remembering events and facts. Also called explicit memory.
Memory of factual information
Memory of an autobiographical event
conversion of short term memory into long term memory
memory of how to do something
decrease in response after repeated presentation of a stimulus
increased effect of a drug after repeated administration
association of a stimulus with a cue such as with classical Pavlovian conditioning
storing information temporarily while simultaneously manipulating those pieces of information
a memory trace
Inability to create new declarative memories
Inability to retrieve memories from one's past
Type of unconscious memory that allows for remembering procedures and performance of specific tasks