31 Olfactory System
Olfaction is the ability to sense and perceive volatile chemicals that are suspended in the air. The typical human can distinguish up to 10,000 distinct odors, ranging from the sweet aroma of esters produced by apples and oranges, to the putrid smells of sulfurous compounds produced by skunks and rotten eggs. Because odors can drift along in the air, some chemicals can be detected long before the source is within eyesight: think about smelling a burning bonfire from miles away.
Smells affect our conscious behavior. They can motivate us to approach freshly-baked bread or avoid a rotting animal carcass. These chemicals serve as survival cues: bread gives us energy-rich carbohydrates while a decaying carcass can expose us to disease.
Smell is often used in communication between animals, especially in reproductive behaviors, territory marking and identification of individuals. Olfaction is one of the oldest functions we possess as animals. While our sense of smell has seemed to take a backseat to other senses relative to other animals (about 2% of the total mouse brain mediates smell, while only a scant 0.01% of the volume of the human brain is dedicated to this function), scientists now appreciate that our olfactory system is simply more specialized. For example, humans use the smells of sweat to clue us into the emotional state of others, and we can even subconsciously detect sickness through body odor.
Anatomy of the Olfactory System
Your sensation of smell begins when odorant molecules travel through your nostrils and pass through the nasal cavity, an empty, air-filled space just behind the front of the skull.
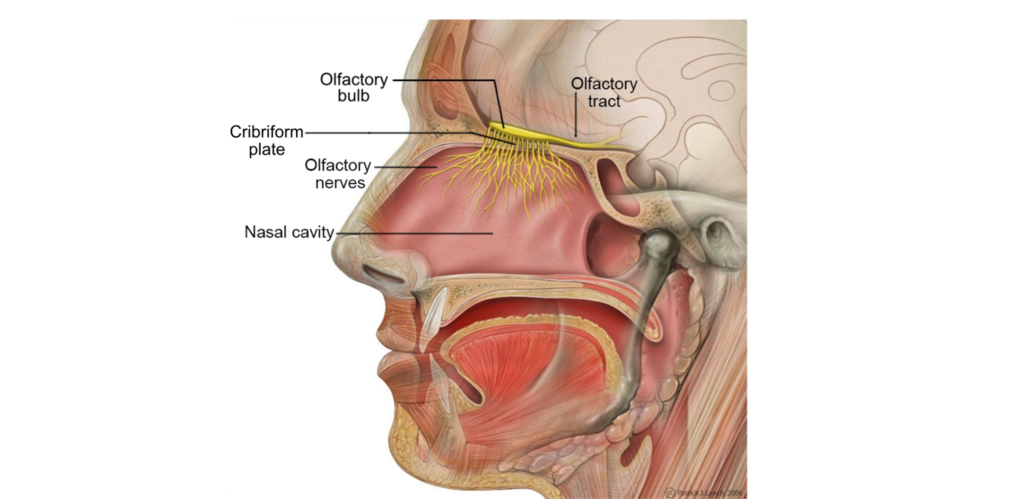
The odorants dissolve in the mucus covered dorsal-most portion of the nasal cavity, an area called the olfactory epithelium. Embedded within the olfactory epithelium are olfactory receptor neurons, supporting cells, and basal cells.
The axons of the olfactory receptor neurons travel through a sheet of bone called the cribiform plate before going to the olfactory bulb. Collectively, the axons of the olfactory receptor neurons make up Cranial Nerve 1 (Olfactory Nerve).
Also found within the olfactory epithelium and surrounding the olfactory receptor neurons are supporting cells and basal cells. The supporting cells help dispose of dead and dying cells, metabolize pollutants, and may also help to physically maintain the epithelium by producing mucus. The basal cells function to replace the olfactory receptor neurons.
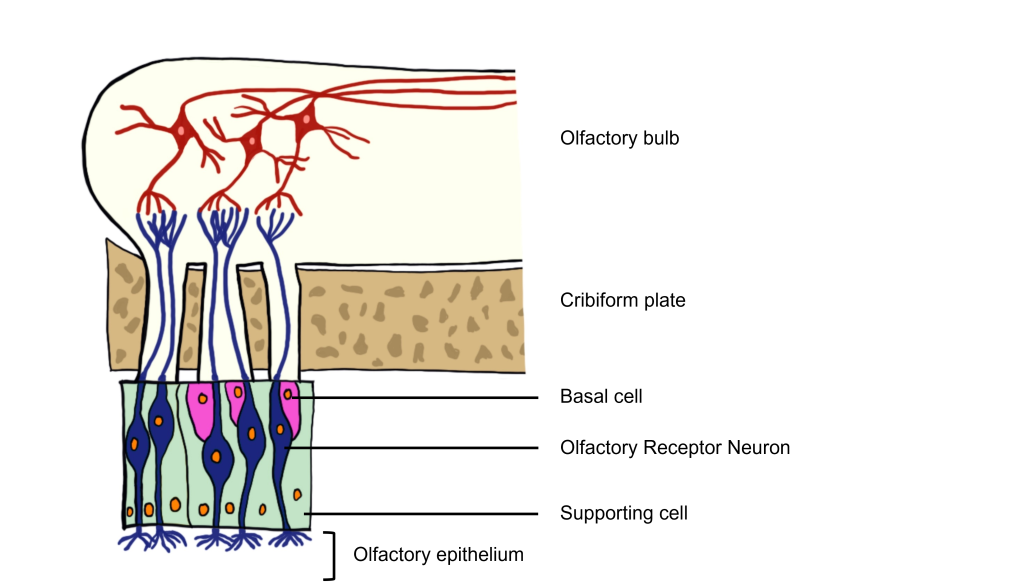
Olfactory Receptor Neurons
The olfactory receptor neurons begin processing smell. They serve as the sensory neurons for the olfactory system. Olfactory receptor neurons are bipolar neurons, that have a single dendrite with many cilia that are exposed to the epithelial surface. The cilia stretch into the mucus of the olfactory epithelium and act to increase the surface area of the neurons, providing increased space for odorants to interact with the olfactory receptor neurons. When the odorants dissolve in the mucus they interact with odorant receptor proteins on the cilia of the olfactory receptor neuron.
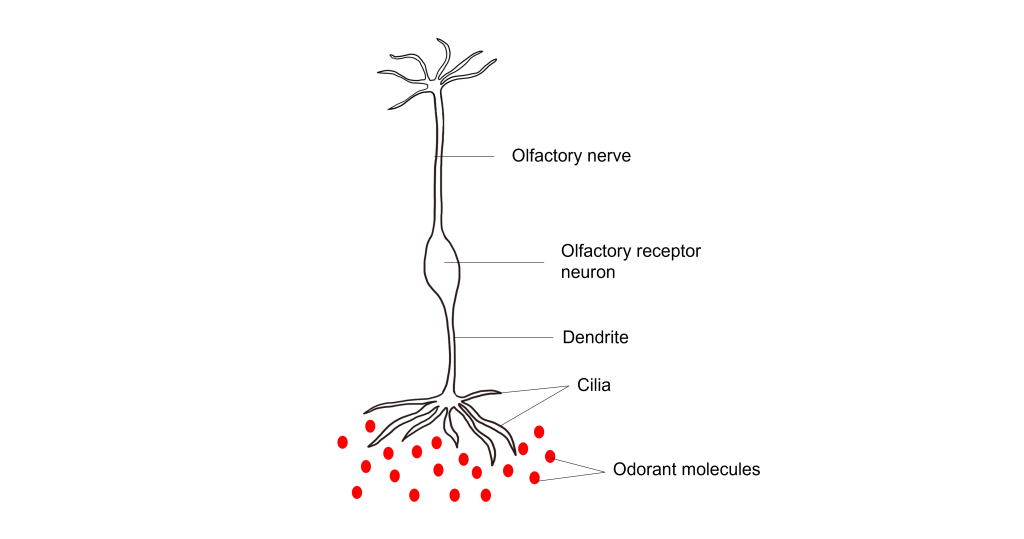
Their proximity to the air make them the only neurons that are directly exposed to the outside world. Unfortunately, this causes them to encounter all sorts of dangers such as toxins, particulates, and microbes. They are one of the few known populations of neurons where adult neurogenesis regularly occurs, each having a lifespan in the range of 30 days to a year. The average human olfactory system has somewhere between 6-20 million olfactory receptor neurons.
Olfactory Transduction
Though taste uses different signaling systems for transduction of each of the different tastes, olfaction uses a single system for transduction. First, odorants bind to odorant receptor proteins (a G-protein- coupled receptor) on the cilia of the olfactory receptor neurons.
Odorant Receptor Proteins
It is estimated there are about 1,000 different genes (about 3% of the total human genome) that code for roughly 400 different odorant receptor proteins[1]. Each olfactory receptor neuron expresses only one type of odorant receptor protein. The initial research into the genetics underlying these neurons helped reveal the mechanism underlying olfactory transduction and specificity, and earned Drs. Linda Buck and Richard Axel a Nobel prize in 2004[2].
Odorant receptor proteins are transmembrane G protein-coupled receptors that have extracellular binding sites on their surface that bind to odorants. Each receptor differs in their specific binding pockets. Importantly, the receptor does not bind to one specific odorant, but rather binds to a specific molecular feature of the odorant. This means that many odorants can activate more than one odorant receptor protein, ultimately allowing each odorant to generate a unique pattern of activity across the olfactory receptor neurons.
Thus, analyzing the activity of a single olfactory receptor neuron is not sufficient to understand how that odorant alters neural signaling. Rather, the olfactory system uses population coding.
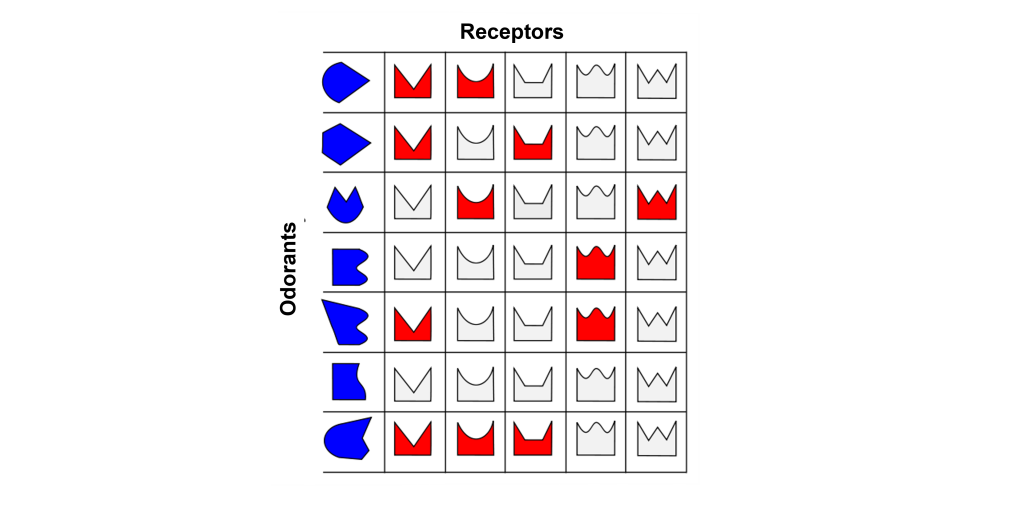
Odorant Receptor Protein Example
In this example we are looking at three different olfactory receptor neurons that express different types of odorant receptor proteins labeled Cell 1 (‘blue’ odorant receptor), Cell 2 (‘orange’ odorant receptor), and Cell 3 (‘purple’ odorant receptor). These neurons are exposed to four different odorants (vanilla, lavender, mint, and rose), and their neural activity is measured via an electrode.
When looking at the response to the ‘Vanilla” odor, cell 1 shows an increase in the rate of action potentials, cell 2 shows very little activity, and cell 3 shows an increase in action potentials. Whereas the ‘Lavender’ odor only causes a response in cell 2. The ‘Mint’ odor causes very little activity in cell 1, and increased activity in both cell 2 and cell 3. Lastly, the ‘Rose’ odor causes an increase in activity in cell 1 and cell 2, but very little activity in cell 3. These recordings demonstrate that a single odorant can cause changes in activity in more than one type of olfactory receptor neuron, due to the receptor proteins recognizing more than one type of molecular feature of the odorant molecules.
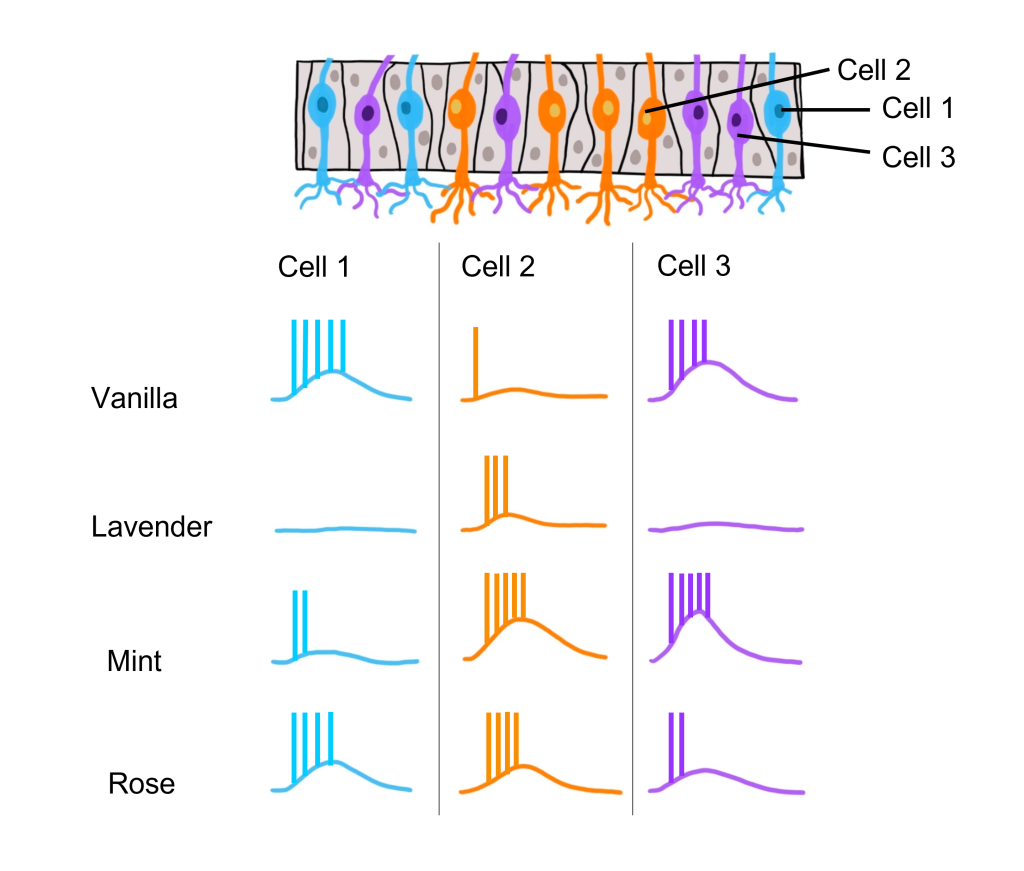
Transduction Pathway
After the odorant binds to the odorant receptor protein, the receptor activates an associated G protein called Golf. This protein complex is 90% similar to the stimulatory G protein Gs , and likewise triggers activation of adenylyl cyclase, elevating the intracellular concentration of cyclic AMP. cAMP binds to cyclic nucleotide-gated cation channels that when opened, allow for the influx of calcium and sodium. The influx of calcium opens calcium-activated chloride channels. Olfactory receptor neurons have high intracellular chloride concentration, so the opening of the chloride channel results in chloride flowing out of the cell. Together, this causes the olfactory receptor neuron to depolarize, leading to membrane depolarization within the dendrite. This change in membrane potential is called a “receptor potential”. If depolarization crosses threshold potential within the olfactory receptor neuron cell body, then the olfactory receptor neuron will fire action potentials down its axons that make up the olfactory nerve toward the olfactory bulb.
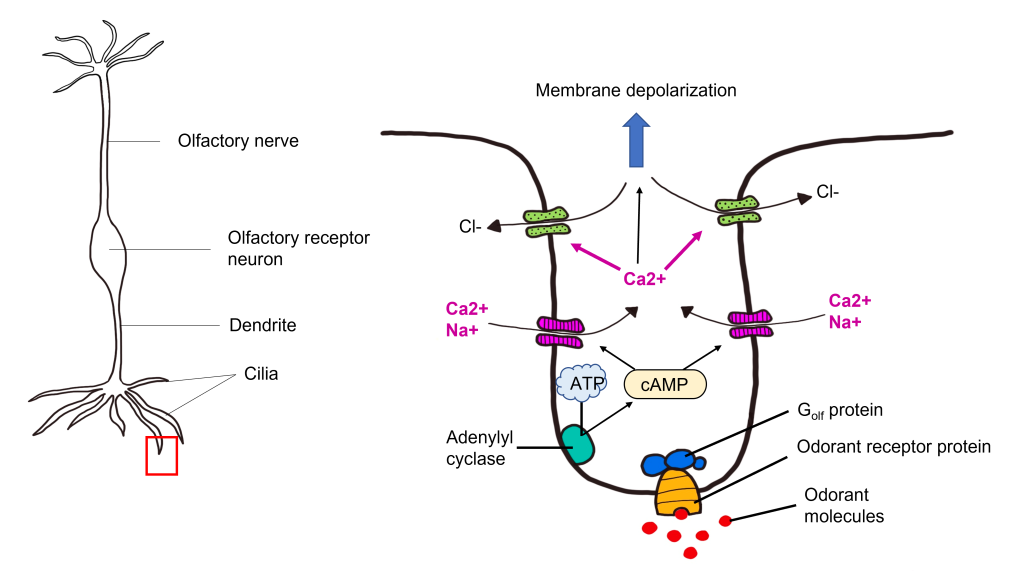
Olfactory receptor neurons encode the intensity of smells through the frequency of action potential firing, which changes in accordance with the concentration of odorant molecules. Imagine standing over a fresh-baked pizza and inhaling deeply. Due to the high concentration of odorant molecules in the air, several receptors will be activated, leading to frequent neuronal firing. Now, imagine you are down the block from a pizza restaurant, getting only a slight whiff of those same scents. Here, the concentration of odorants is low, meaning that the Olfactory receptor neurons fire less frequently.
Termination of Olfactory Signaling
Because odorants are found in the air, they are highly mobile and can easily diffuse away, no longer having the ability to activate odorant receptor proteins. Olfactory signaling can also be terminated through the activity of enzymes located within the mucus of the olfactory epithelium.
Our perception of smells can also fade, even if the odorant is still present in the air. The odorant receptor neurons can adapt to the presence of an odorant, and thus no longer signal its presence.
Central Olfactory Pathway
The axons of the olfactory receptor neurons pass through the skull through a tiny series of holes at the cribriform plate. These primary neurons form synaptic connections onto neurons in the olfactory bulb called mitral cells, forming the beginning of the olfactory nerve (CN I). Like the optic nerve, the olfactory nerve runs along the ventral surface of the brain.
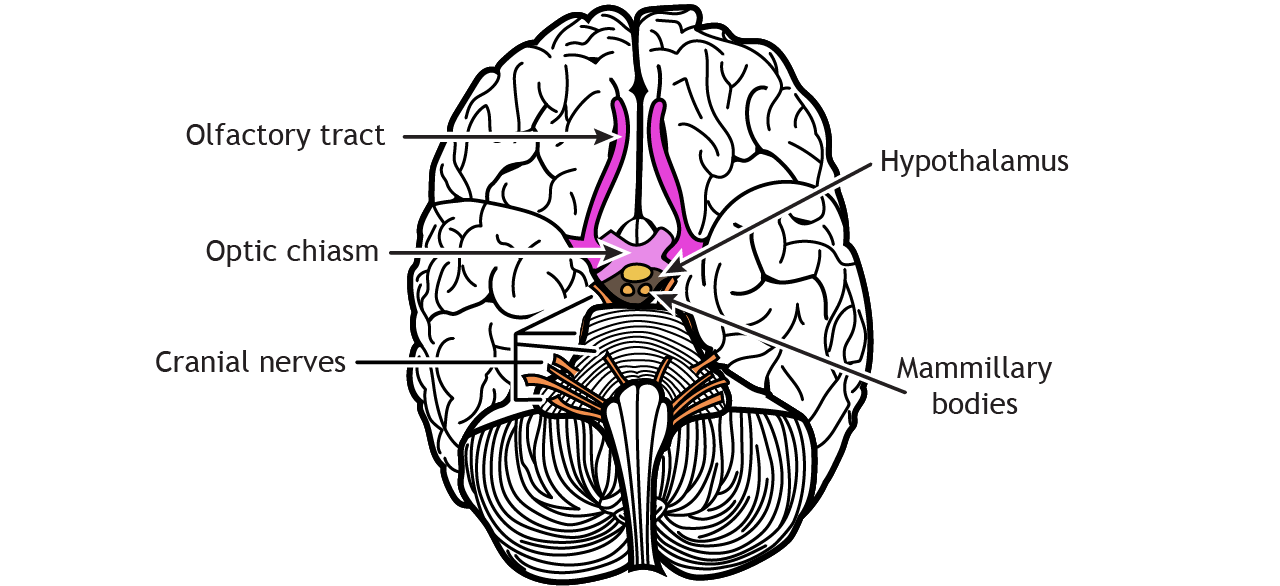
Glomeruli within the Olfactory Bulb
The site of synaptic connectivity between the olfactory receptor neurons and the secondary neurons in the olfactory bulb (mitral cells) is a highly specialized clump of tissue called a glomerulus. The typical human has a little under 2,000 glomeruli, and each glomerulus only receives inputs from olfactory receptor neurons that express the same type of odorant receptor proteins. This means that even through the olfactory receptor neurons that express the same type of odorant receptor protein are spread out throughout the olfactory epithelium, the axons of those neurons all converge within the same glomerulus.
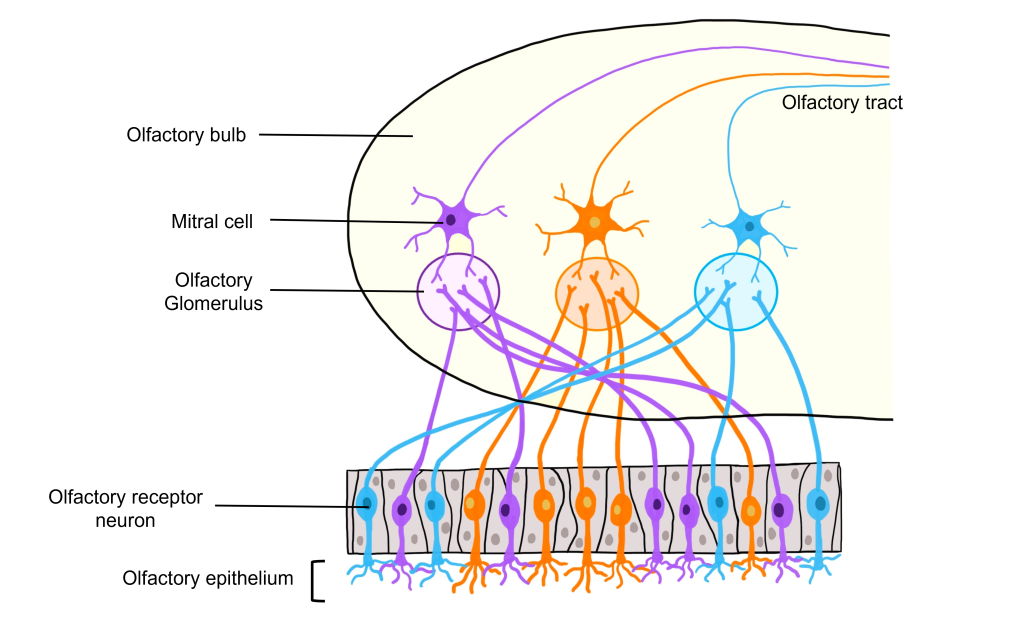
Brain Targets of Olfactory Neurons
Axons leave the olfactory bulb via the olfactory tracts and project to two different targets.
First, some cells project to the olfactory tubercle, are routed through the medial dorsal nucleus of the thalamus and then to the orbitofrontal cortex. This pathway is responsible for our conscious perception of smell.
Other cells project directly to the olfactory cortex and other related structures found within the temporal lobes, like the hippocampus. This makes the olfactory system the only sensory system that does not have to first pass signals through the thalamus before cortical processing. This pathway may play roles in discriminating odors, and the emotional, motivational, and memory-related aspects of smell.

Disorders of the Olfactory System
Like other sensory systems, the structures involved in olfaction can be injured. An injury to the olfactory system can result in hyposmia, a reduced ability to smell, or anosmia, a complete loss of smell.
The most common insult to the olfactory system is simple nasal congestion, a temporary, physical blockage of the entry to the nasal cavity that decreases airflow and, therefore, the number of particles that reach the olfactory epithelium. Congestion can be caused by allergies, the common cold, upper respiratory bacterial or viral infections, or sinus infections.
Hyposmia is also one of the main neurological symptoms of COVID-19. Hyposmia is common among healthy, older adults, affecting about half of the population between 65 and 80 years old. As a person ages, spontaneous calcification causes the holes in the cribriform plate to shrink, which can impinge on and damage olfactory receptor neuron axons. Hyposmia can also be caused by abrupt head injuries. The olfactory receptor neuron axons that project through the holes of the cribriform plate are particularly sensitive to blows to the head. Neurodegenerative disorders, such as Parkinson’s disease and Alzheimer’s disease, contribute to smell deficiency. Usually, hyposmia precedes the major clinically observed symptoms of these disorders, hinting that smell deficiency may serve as an early diagnostic biomarker.
Another olfactory deficit, phantosmia, is when a person perceives “phantom” scents, or in other words, experiences an olfactory hallucination. Phantosmia may be triggered by a temporal lobe seizure or a stroke. It can also be caused by a brain tumor affecting the olfactory nerve (CN I), or the subsequent surgical removal of the tumor, leading to injury. Schizophrenia, a psychiatric condition characterized by auditory hallucinations, may also cause phantosmia.
Key Takeaways
- Odorants dissolve in the mucus of the olfactory epithelium and bind to odorant receptor proteins on olfactory receptor neurons.
- A single odorant can bind to multiple different odorant receptor proteins because the odorant receptor protein binds to a molecular feature of the odorant.
- Odorant receptor proteins are G protein-coupled receptors that cause accumulation of cAMP within the cell, and ultimately membrane depolarization of the olfactory receptor neuron.
- Olfactory information is carried from the olfactory receptor neuron axons through the cribiform plate and into the olfactory bulb where it synapses with the olfactory glomeruli. From there, the information travels through the olfactory tracts and can either be routed through the thalamus and then to olfactory brain areas, or can be directly routed to olfactory cortical areas, bypassing the thalamus.
Test Yourself!
Attributions
This chapter is adapted from “Olfactory System” in Introduction to Neuroscience by Valerie Hedges which is licensed under a Creative Commons Attribution-NonCommercial-ShareAlike 4.0 International License.
Media Attributions
- Olfactory system © Patrick J. Lynch is licensed under a CC BY (Attribution) license
- Close up of olfactory receptor neuron © Valerie Hedges is licensed under a CC BY-NC-SA (Attribution NonCommercial ShareAlike) license
- olfactory receptor neuron © Valerie Hedges is licensed under a CC BY-NC-SA (Attribution NonCommercial ShareAlike) license
- odorant proteins © Joanna Kośmider adapted by Valerie Hedges is licensed under a Public Domain license
- odoran receptor example © Valerie Hedges is licensed under a CC BY-NC-SA (Attribution NonCommercial ShareAlike) license
- Olfactory transduction © Valerie Hedges is licensed under a CC BY-NC-SA (Attribution NonCommercial ShareAlike) license
- Glomerulus © Valerie Hedges is licensed under a CC BY-NC-SA (Attribution NonCommercial ShareAlike) license
- Olfactory pathway © Valerie Hedges is licensed under a CC BY-NC-SA (Attribution NonCommercial ShareAlike) license
A mucus covered area located in the dorsal-most portion of the nasal cavity where odorants interact with olfactory receptor neurons.
Bipolar neurons have two extensions off the cell body
hairlike vibrating structure found in large numbers on the surface of certain cells that cause currents in the surrounding fluid
The process where new neurons are generated
A G-protein coupled receptor located on olfactory receptor neurons
When the membrane potential gets closer to zero
Toward the bottom of the brain or the front of the spinal cord
reduced ability to smell
a complete loss of smell
olfactory hallucinations